Silicon-based materials hold immense potential for revolutionizing lithium-ion batteries. They offer significantly higher energy storage capacity and a favourable voltage range, making them prime candidates for powering the next generation of devices. However, their practical application is hindered by several challenges, primarily related to their dramatic volume changes, low conductivity, and incompatibility with standard battery liquids.
1. The Allure of Silicon: Unmatched Storage Capacity
Silicon’s theoretical capacity to store lithium is remarkable, exceeding that of conventional graphite by a significant margin. This translates to the potential for lithium battery systems with much higher energy density, allowing devices to operate for longer periods on a single charge.
Moreover, silicon’s voltage characteristics are advantageous. It operates at a voltage that minimizes the risk of unwanted lithium buildup on the electrode surface during charging, enhancing safety.
Furthermore, silicon is abundant and readily available, making it a cost-effective material.
2. The Challenges of Silicon: Overcoming Obstacles
Despite its advantages, silicon faces significant hurdles:
- Drastic Volume Changes: During charging and discharging, silicon undergoes massive volume fluctuations, exceeding 300%. This expansion and contraction can cause the electrode material to detach from the current collector and fracture, leading to rapid capacity loss.
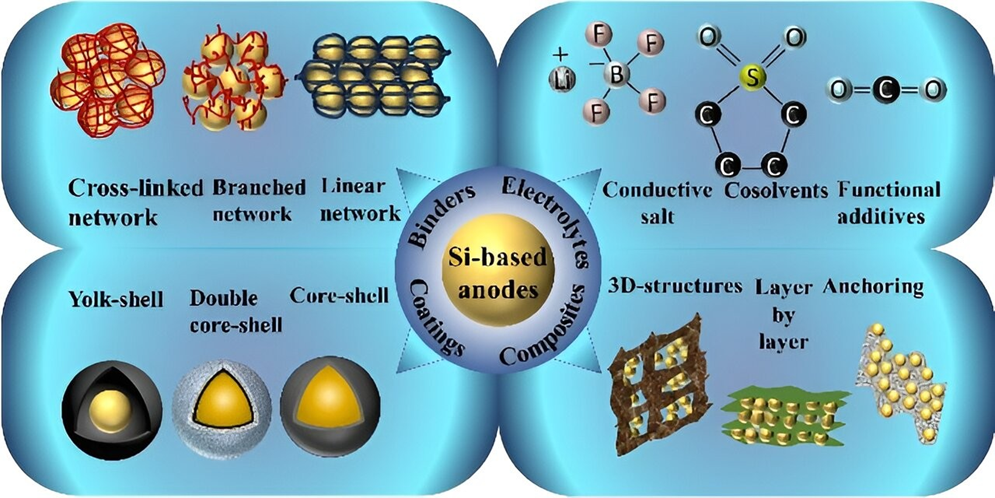
- Low Intrinsic Conductivity: Silicon is a poor conductor of electricity, hindering the efficient flow of electrons. To improve the electrode’s conductivity, conductive additives must be added.
- Unstable Protective Layer: The protective layer that forms on the silicon surface, known as the SEI film, is often unstable. This instability leads to continuous reactions with the battery’s liquid, consuming the liquid and resulting in rapid capacity decay.
3. The Breakdown: Understanding Capacity Fading
The rapid capacity fading of silicon-based electrodes is a major obstacle to their widespread adoption. Several factors contribute to this:
- Electrolyte Decomposition: The standard battery liquid can react with silicon, leading to the formation of an unstable SEI film.
- Structural Degradation: The significant volume changes during cycling cause the silicon particles to break down and lose contact with the conductive network.
- Loss of Electrical Contact: The repeated expansion and contraction of silicon create gaps between particles, disrupting the electrical pathways and reducing capacity.
4. The Path Forward: Strategies for Improvement
To overcome these challenges, researchers are exploring various strategies:
- Material Design: Engineering the structure and composition of silicon-based materials to minimize volume changes and enhance conductivity.
- Electrolyte Development: Developing battery liquids that are compatible with silicon and promote the formation of a stable SEI film.
- Binder Optimization: Designing binders that can accommodate the volume changes of silicon and maintain electrical contact.
- Electrode Architecture: Exploring new electrode designs that can accommodate the expansion and contraction of silicon.
- Nanoscale Engineering: Using very small particles to allow for volume expansion without cracking.
- Composite Materials: Combining silicon with other materials like carbon to provide structural support and conductivity.
While pure silicon electrodes are not yet commercially viable, ongoing research and development efforts, including advancements in lithium battery-making machines and lithium-ion battery-making machines, are paving the way for the realization of high-capacity silicon-based batteries.